Hola amigos : A VUELO DE UN QUINDE EL BLOG., nos es muy grato difundir la noticia del descubrimiento de la Partícula de Bosón de HIGGS, por la Organización Europea para la Investigación Nuclear (CERN).
CERN Press Release: CERN experiments observe particle consistent with long-sought Higgs boson
Geneva, 4 July 2012. At a seminar
held at CERN today as a curtain raiser to the year’s major particle
physics conference, ICHEP2012 in Melbourne, the ATLAS and CMS
experiments presented their latest preliminary results in the search for
the long sought Higgs particle. Both experiments observe a new particle
in the mass region around 125-126 GeV.

“We observe in our data clear signs of a new particle, at the level of 5
sigma, in the mass region around 126 GeV. The outstanding performance
of the LHC and ATLAS and the huge efforts of many people have brought us
to this exciting stage,” said ATLAS experiment spokesperson Fabiola
Gianotti, “but a little more time is needed to prepare these results for
publication.”
"The results are preliminary but the 5 sigma
signal at around 125 GeV we’re seeing is dramatic. This is indeed a new
particle. We know it must be a boson and it’s the heaviest boson ever
found,” said CMS experiment spokesperson Joe Incandela. “The
implications are very significant and it is precisely for this reason
that we must be extremely diligent in all of our studies and
cross-checks."
“It’s hard not to get excited by these results,”
said CERN Research Director Sergio Bertolucci. “ We stated last year
that in 2012 we would either find a new Higgs-like particle or exclude
the existence of the Standard Model Higgs. With all the necessary
caution, it looks to me that we are at a branching point: the
observation of this new particle indicates the path for the future
towards a more detailed understanding of what we’re seeing in the data.”
The results presented today are labelled preliminary. They are based on
data collected in 2011 and 2012, with the 2012 data still under
analysis. Publication of the analyses shown today is expected around
the end of July. A more complete picture of today’s observations will
emerge later this year after the LHC provides the experiments with more
data.
The next step will be to determine the precise nature of
the particle and its significance for our understanding of the universe.
Are its properties as expected for the long-sought Higgs boson, the
final missing ingredient in the Standard Model of particle physics? Or
is it something more exotic? The Standard Model describes the
fundamental particles from which we, and every visible thing in the
universe, are made, and the forces acting between them. All the matter
that we can see, however, appears to be no more than about 4% of the
total. A more exotic version of the Higgs particle could be a bridge to
understanding the 96% of the universe that remains obscure.
“We
have reached a milestone in our understanding of nature,” said CERN
Director General Rolf Heuer. “The discovery of a particle consistent
with the Higgs boson opens the way to more detailed studies, requiring
larger statistics, which will pin down the new particle’s properties,
and is likely to shed light on other mysteries of our universe.”
Positive identification of the new particle’s characteristics will take
considerable time and data. But whatever form the Higgs particle takes,
our knowledge of the fundamental structure of matter is about to take a
major step forward.
What does the news tell us?
With strong evidence of a new
particle with a mass around 126 GeV, will our interpretation of the
Universe change? In an interview with the Bulletin, CERN theorist Ignatios Antoniadis explains.
Is today’s news an answer or the first of a new set of questions about Nature?
Ignatios Antoniadis:
Based on the current data, this new particle looks like the long
awaited Higgs boson. In this respect, the result is an important answer.
However, the properties of the new particle have not been fully studied
yet and therefore a final label will only come after further
investigations.
The next step will be looking into the new
particle’s decay rates. Together with mass, decay rates characterize
particles. If we find that this new particle decays in the ways expected
and with the rates expected from the Standard Model, we will be able to
really identify it with the particle responsible for the electroweak
symmetry breaking (*).
Otherwise?
Antoniadis:
Otherwise we will have to understand whether the Standard Model needs
small adjustments to include the existence of the new particle or if new
physics processes need to be looked for in order to explain its nature.
Let’s stay with the Standard Model. What is the new particle telling us?
Antoniadis:
If the new particle is confirmed to be a Standard Model Higgs boson,
then we must observe that it is relatively light, perhaps lighter that
what a large part of the community was expecting up until just a few
months ago.
The Higgs field associated with the boson would
still permeate the Universe but it may need to be partly reinterpreted.
Given the low mass of the boson, the potential that describes the field
could for instance present two minima instead of just one. The universe
is currently set in one of the two minima but quantum mechanics could
allow for a transition to the second minimum. This, in my opinion, might
signal the existence of some new physics that would compensate for this
instability (**).
Has the current result already ruled out some previously possible descriptions of the Universe?
Antoniadis:
Yes. Because of its low mass, such a Higgs boson would allow us to rule
out theories known as “Technicolor” and some of the theoretical models
used in Supersymmetry. However, other supersymmetric or not scenarios
could still apply, as well as extra-dimensional theories.
Let’s now leave the Standard Model for a moment. What is the new particle telling us?
Antoniadis:
The new particle could have properties that are not those predicted by
our current theories. In this case, in order to unveil the theoretical
scenarios to fit the new description of the Universe we would need to
know exactly what its decay modes and rates are. The good news for
theorists is that experimentalists may be able to provide us with this
information over the next a few months. Our (and your) curiosity will
soon be satisfied!
This result opens a new era of physics with new possible discoveries likely to come.
For articles by Peter Higgs, Robert Brout, François Englert, John Ellis, Giovanni Ridolfi, Fabio Zwirner, Ignatios Antoniadis and other scientists, please visit: http://www.sciencedirect.com/science/journal/16310705/8/9
This result opens a new era of physics with new possible discoveries likely to come.
For articles by Peter Higgs, Robert Brout, François Englert, John Ellis, Giovanni Ridolfi, Fabio Zwirner, Ignatios Antoniadis and other scientists, please visit: http://www.sciencedirect.com/science/journal/16310705/8/9
(*) Please read FAQ n. 2.
(**) For a recent study of the possible instability of the Higgs potential, see: http://arXiv.org/pdf/1205.6497.pdf
Interview by Antonella Del Rosso
It’s no miracle...
“The LHC has been a huge effort
over more than 15 years. It’s not always been easy but today it is
performing so spectacularly well that the people who built it should be
very proud.” Lyn Evans, head of the LHC project since its beginning and
until its commissioning in 2011, shares his recollections with us on the
day of the long-awaited discovery.
While you were building the LHC, were you expecting it to perform so well?
Lyn Evans: I
knew that the machine had been beautifully designed and was being built
extremely well – but I could not have expected that such wonderful
results could be achieved in so little time.
What are the reasons for such a good performance?
Evans: The
LHC was designed to really push the limits; I think that the machine
has got more than 30 years of accumulated experience in its design. The
fact is the LHC has already exceeded the design luminosity at the energy
it’s at now. At 4 TeV it should be at 5 x1033 – and it is quite a bit higher.
So, the LHC has exceeded expectations – and the reason is because of
the quality of the design. There are no miracles; this is why the
machine has been working so incredibly well. The quality of the magnets
and the stability of the power supplies are fantastic – much better than
has ever been achieved before. And the quality of the instrumentation
is essential - the LHC is instrumented like nothing ever before. Even
during the financial crisis in 2001, when there was a lot of pressure to
reduce cost, we never gave in a single centime for instrumentation.
A financial crisis that was about to jeopardize the whole project…
Evans: When
the project started we said that we would review the cost at mid-term
and adjust accordingly. When we did that, we found it 18% higher than
the 1993 cost projection. This, on such a technically advanced project
that had never been built before, could be regarded as a relatively
small variation. However, it did indeed cause a lot of reactions.
What are the challenges that you had to face?
Evans:
The challenges changed over time. In the first few years, they were
essentially political. The challenge was with getting approvals, and
getting some big countries on board (the non-Member States). Then, of
course, the challenge became development and design. When we got the
approval from the CERN Council, we didn’t have a single magnet that
worked. After that, the challenge became massive manufacturing, where we
had lots of problems. You will certainly remember, for instance, issues
with the cryogenic line.
Of course the real kick in the teeth
was the last one, when we were taking the very last sector up to high
current and a joint failed. It caused a lot of pain, although we did
have spare parts, which was the most important thing.
So, all
the way along the challenges have been different. I had to face 5
bankruptcy cases – and, I can tell you, that’s not funny. When you’re in
the middle of production and suddenly the firm goes bust, how do you
react? So – let’s say – there was a whole spectrum of things that had to
be dealt with.
And we all remember the commissioning – the day
of the first beam in the LHC – when the pressure was absolutely immense.
But in one hour we had the machine beam circulating, and I’d never seen
anything like it before. Normally, this would have taken 2 weeks!
You
were recently appointed Linear Collider Director by the International
Committee for Future Accelerators. Cutting-edge accelerators are still
inspiring you!
Evans: Well, I came to CERN as a
laser physicist, not as a HEP physicist. I first came to CERN in October
1969 for 3 months because they were working with high power lasers. And
then I got a fellowship and – just by chance – became involved in
building an experimental linear accelerator. That was my first taste of
accelerator physics. It was a 3 MeV accelerator which was basically the
prototype for LINAC2 and LINAC4.
In 1971, I started working on
building the SPS. And, after the idea of stochastic cooling had been
proved in the late 70s, I worked on converting the SPS into a
proton-proton collider, all the way through to the Nobel Prize in 1984. I
also worked for a while at Fermilab’s Tevatron, so I learned about
superconducting machines there. I had a relatively short spell – 4
years, from 1990 to 1993 – as division leader of the SPS LEP division.
And I was responsible for running and developing LEP, after which I took
on developing the LHC. In 1996 we got full approval. I was LHC project
leader the whole time, until the commissioning of the machine. So, the
LHC has been a big part of my life.
I am lucky to have been
involved in all the big projects here at CERN – and I guess that’s the
way you learn. Of course, you can’t help but be motivated when you are
working in such a fantastic place.
What do you think is the future of the LHC after the Higgs?
Evans: I
think that it has a long future. There is nothing like it and there
will be nothing like it for a very long time. Even if a linear collider
is approved in the next 3 or 4 years, it is going to take around 15
years to build. Furthermore, the LHC is now the only instrument that can
access very high energies. I think the LHC is going to be developed to
its full capability – both the machine and its detectors will be
improved over the coming years. The detectors are already a marvel and
the way that they perform is incredible.
I hope that there will
be many discoveries coming up for the LHC – the Higgs is just the first
step. I think it is CERN’s job – independent of whether we build a
linear collider or not – to exploit the LHC to its full potential. It
may be that science will tell us that we need a higher energy LHC –
which today would be very, very difficult to build. But there is still
R&D going on – one could imagine doubling the LHC energy, for
instance. We build accelerators because science tells us that that is
what we need to make achievements possible.
Anyway, I think the
LHC works so well because of the people: the people who designed it and
built it, and also the people who are running it right now, who are just
a fantastic group.
.. It’s a blooming miracle - a special LHC Report
Wrestling a 27 km superconducting
collider under control is not easy. Throw in high intensity beams and it
can sometimes seem a continual, frustrating battle with the vagaries of
a hugely complex problem space.
This problem space
has been thoroughly explored over the last three or four years. The
myriad of potential obstacles to smooth operation ranges from
unidentified falling objects (UFOs), electron clouds, beam dynamics,
radiation to electronics, vacuum instabilities, “transparent” software
changes, Radio Frequency (RF) trips, electrical network glitches etc.
etc. The huge extended systems, such as the beam loss monitors,
cryogenics, and quench protection systems, have a phenomenal number of
components that inevitably have occasional failures (there is, of
course, a higher probability that these occur late Friday evening and
over the weekend). On the cryogenics front, cooling and keeping 36,000
tonnes of magnets at 1.9 K is a challenging prerequisite for everything
else that follows.
Despite their complexity, we have very well
behaved magnets and overall the machine is stable and magnetically
reproducible. The magnets are well understood following a long and
careful measurement campaign carried out during production. A
sophisticated magnet model is even capable of dealing with the once
feared dynamic effects. Together with the accuracy and stability of the
power converters, our carefully optimized machine stays optimized. The
injection, ramp and squeeze have been mastered and, as a general rule,
injected beam makes it into collisions.
Exploitation of the
LHC’s potential is helped by the presence of excellent beam
instrumentation and powerful high-level software architecture. Together
with some applied intelligence, these have allowed the development of
tools (e.g. measurement and correction of optics, an on-line aperture
model) which have opened the way to maximizing the performance of the
machine. Most notably, accurate measurements of the aperture in the
regions adjacent to the experiments have allowed us to reduce the beam
size at the interaction points to unexpectedly low values. The
better-than-expected aperture reflects good observance of tolerances
during installation and very good alignment of all elements by the
survey group.
The LHC has enjoyed from the start very good beam
quality (both protons and ions) from the injector complex. The bunch
currents have been well above, and the beam sizes well below, the
nominal values quoted in the design report. The production of beam in
the LINAC, Booster, PS, and SPS is distinctly non-trivial and requires
continual care and attention to maintain the beam parameters, however
this diligence is reflected directly in delivered luminosity.
Naturally, a cautious approach marked the re-starting in November 2009
following recovery from the 2008 incident. This was most clearly
reflected in the choice to run at an initial beam energy of 3.5 TeV.
Having experienced first hand the destructiveness of magnetic energy,
awareness of the damage potential of the beam to the machine has
underpinned the operational approach and marked the subsequent evolution
in beam intensity. The full and proper functioning of the extended
machine protection system (MPS) has always been an absolute priority.
The MPS consists of a federation of inputs from various systems into a
beam interlock system (BIS). When the BIS is triggered it provokes a
beam dump within 3 to 4 turns (that is, in a few hundred millionths of a
second). The MPS has worked flawlessly, always pulling a beam abort
when called upon to do so.
In addition to the MPS, the beam
drives a subtle interplay of the beam dump system, the collimation
system and protection devices, all of which rely on a well-defined
aperture, orbit and optics for guaranteed safe operation. Assuring this
throughout high intensity operation remains paramount. Numerous
interlocks are in place to ensure that posted limits are always
respected.
One notable feature during LHC commissioning and
operation is the collective ability of CERN teams to resolve problems.
There is in-depth expertise and experience on all systems, including
vacuum, collimation, RF, fast kicker magnets and so on. Serious issues,
such as radiation to electronics, and the lack of redundancy in
protection systems, are targeted rigorously as they become apparent.
Although precision and rigour are needed when dealing with the tightly
synchronized choreography and the ever present dangers of magnetic and
beam energy, an open and mostly friendly atmosphere pervades. A recent
visitor to an 8:30 meeting noted the lack of defensiveness, and a
willingness to directly engage a problem without needing to assign
blame. There is amazing dedication from everybody involved. Problems
that stop the operation of the machine happen anytime, with a slight
preference for nighttime and the weekend. Despite this, there is
unfailing support from all teams. Coming out of the technical stop this
last weekend the Machine Protection and Electrical Integrity team worked
until 5 in the morning on Saturday, and the control and timing teams
were in from 2 to gone 6 on Sunday morning dealing with the effects of
an innocent leap second.
Finally, trying to hold everything
together across the accelerator complex is a talented, smart, moderately
good-looking operations team who have necessarily developed an advanced
sense of humour.
The LHC came out of a five-day technical
stop on the evening of Friday 29 June. For real-time information on the
operation of the machine, visit the LHC Page 1 status page.
A landmark day (not only) in CERN's history
Today, the ATLAS and CMS
experiments announced that they had observed a new particle. We don’t
yet know what that particle is, but it is consistent with the long
–sought Higgs boson, and work will soon be underway to positively
identify it. Days like this do not come around very often, and it’s a
cause for celebration.
Today we are privileged to be joined by many important guests,
including some of the early authors of electroweak symmetry breaking:
Peter Higgs, François Englert, Gerry Guralnik and Carl Hagen. We also
have around 100 representatives of the media here to cover the event,
and finally lay to rest the speculation and anticipation that has been
building over the last few weeks.
Now that we have the
discovery, we need to thoroughly investigate all the properties of this
new particle to establish whether it is the Higgs boson that completes
the Standard Model, or something more exotic. The Standard Model is a
beautiful theory that accounts for the fundamental particles that make
up the visible universe, and all their interactions with the exception
of gravity. We know, however, that the visible universe is only about 4%
of the total. A more exotic version of the Higgs particle could be a
bridge to understanding the 96% of the universe that remains obscure.
The fact that we have reached this point so quickly has come as a
surprise even to me, and it’s thanks to the incredible work of everyone
involved in the LHC programme. Your efforts have delivered the data,
processed and analysed them with efficiency unthinkable just one
generation of experiments ago. The LHC and all the infrastructure that
supports it continue to perform beyond all expectations. Analyses are
refined at a breathtaking rate: it’s been fascinating to see how the
signal at 125-126 GeV has evolved over the last 12 months from a whisper
to a shout. And the Worldwide LHC Computing Grid continues to take all
this comfortably in its stride.
I’d like to thank all of you for
today. I am no longer directly involved in the research, but thanks to
your efforts, I have a most privileged position to see science history
in the making.
Rolf Heuer
Frequently Asked Questions: The Higgs!
Why have we tried so hard to find
the Higgs particle? How does the Higgs mechanism work? What is the
difference in physics between strong evidence and a discovery? Why do
physicists speak in terms of "sigmas"? Find out here!
- Why have we tried so hard to find the Higgs particle?
Because it could be the answer to the question: how does Nature decide whether or not to assign mass to particles?
All the fundamental particles making up matter – the electron, the quarks, etc. – have masses. Moreover, quantum physics requires that forces are also carried by particles. The W and Z particles that carry the weak force responsible for radioactivity must also have masses, whereas the photon, the carrier of the electromagnetic force, has no mass at all. This is the root of the “Higgs problem”: how to give masses to the fundamental particles and break the symmetry between the massive W and Z and the massless photon? Just assigning masses by hand leads to an inconsistent theory and nonsensical predictions. Nature must therefore have a way of correcting this inconsistency, and the mechanism proposed by Englert, Brout and Higgs could be the answer. |
- How does the Higgs mechanism work?
According to the Englert-Brout-Higgs mechanism, the property that we
measure as the ‘mass’ of a particle is the result of a constant
interaction with a field that permeates the Universe like a sort of
“ether”. The existence of this Englert-Brout-Higgs field is definitively
proven by the discovery of the corresponding quantum particle - the
Higgs boson.
Originally, the Englert-Brout-Higgs mechanism was
put forward to explain why one of Nature’s fundamental forces has a very
short range, whereas another similar force has an infinite range. The
forces in question are the electromagnetic force (infinite range) –
which carries light to us from the stars, drives electricity around our
homes, and holds together the atoms and molecules from which we are all
made – and the weak force (very short range), which is responsible for
radioactivity and drives the energy-generating processes of the stars.
Today we know that the electromagnetic force is carried by particles
called photons, which have no mass, whereas the weak force is carried by
particles called W and Z, which do have mass. Rather like people
passing a ball, interacting particles exchange these force carriers. The
heavier the ball, the shorter the distance it can be thrown – and the
heavier the force carrier, the shorter its range. The W and Z particles
were discovered in a Nobel prize winning enterprise at CERN in the
1980s, but the mechanism that gives rise to their mass had not yet been
understood, and that’s where the Higgs boson comes in.
The
Englert-Brout-Higgs mechanism in its basic form is the simplest
theoretical model that could account for the mass difference between
photons and the W and Z particles, and by extension could account for
the masses of other fundamental particles. The presence of the
Englert-Brout-Higgs field enables these forces to cohabit a single
unified electroweak theory.
It should not be thought that the
Englert-Brout-Higgs field is responsible for all the mass in the
Universe. Your interaction with the field actually contributes less than
1 kg to your mass. The remainder comes mainly from the strong force
binding quarks inside nucleons, with a tiny contribution from the
electromagnetic force that reigns over the atomic and molecular scales.
Higgs bosons are quantum fluctuations in the Englert-Brout-Higgs field that are visible experimentally only when energy is “injected” into the field. Concentrating the right amount of energy in proton-proton collisions at the LHC excites the Englert-Brout-Higgs field, which resonates at a precise energy corresponding to the mass of the Higgs boson. The Higgs boson appears momentarily before decaying into other particles that the LHC experiments can measure. Some theories predict the existence of multiple Higgs bosons. |
- Is the Higgs boson the only possible answer to the “mass problem”?
No, there are other theories that predict the existence of different
mechanisms to explain how Nature deals with the mass problem. For
example, there are rival theories that suggest the existence of extra
dimensions of space.
Also, despite the fact that we see strong
evidence of its existence, we do not yet know whether the Higgs boson is
an elementary particle as postulated in the Standard Model, or some
more complex object. Nor do we know whether there is only one Higgs
boson or if there are more of them. Further studies and analysis will
have to be carried out to reply to these questions.
- Why is it called the “God particle”?
The term was coined for Leon Lederman's popular science book on particle physics: “The God Particle: If the Universe Is the Answer, What Is the Question?”
- Is Peter Higgs the only theorist who proposed this mechanism as a solution to the “mass conundrum”?
No. In 1964 independently and almost simultaneously, The theory of the
Higgs field was proposed by three groups of physicists: François Englert
and Robert Brout, Peter Higgs, and Gerald Guralnik, C. R. Hagen, and
Tom Kibble. However, Peter Higgs was the only one of these who pointed
out explicitly the existence of the particle that bears his name and
calculated some of its properties.
- What is the difference in physics between “strong evidence” and a discovery? Why do physicists speak in terms of “sigmas”?
The Higgs boson cannot be observed directly because its lifetime is too
short for our apparatus. At the end of its life, the boson decays and
transforms into other particles, and the detectors may detect these
decay products. As an example, one of the ways a Higgs particle can
decay is into two photons, which can then be detected. However, there
are many other processes that also produce two photons, so researchers
compare the number of so-called “two-photon events” measured with the
number expected from known processes. They do this for all the possible
decay modes, and only when they see a statistically significant excess
of events can scientists claim a discovery.
In particle physics,
people talk of 95% confidence levels, which means that a given signal,
such as that for a Higgs particle decaying to two photons, has only a 5%
chance of being due to a statistical fluctuation. However, 95%
confidence is not enough to claim a discovery. For that, the probability
of a statistical fluctuation being responsible for the measurement has
to be much smaller, less than one in a million. This is what physicists
call a five-sigma effect. It is considered the gold standard for
significance; six sigmas correspond to one chance in half a billion that
the result is a random fluke.
- Why did it take so long to come to such a result?
First of all, accelerators have to be powerful enough to produce the
high-energy collisions that allow any given particle to be created. The
lowest energy that you need in a collision in order to create a given
particle is the mass of the particle itself. However, the particle you
are looking for might be produced together with other particles, in
which case a higher collision energy would be needed.
In a
proton-proton collider such as the LHC, the physics processes are such
that the probability to produce a Higgs boson increases considerably
when the energy collision is increased. As an example, the Higgs boson
production rate in 2011 – when the LHC was operated at 3.5 TeV per beam –
was about 27% less than the production rate in 2012, when the LHC is
being operated at 4 TeV per beam.
In general, the processes
associated with the observation of the Higgs boson are very rare, and
therefore statistics come into play. The statistical error, i.e., the
expected range of statistical fluctuations, goes down as the inverse of
the square root of the data sample size. For example, to halve the error
bar you must quadruple the data sample. This is why physicists always
try to collect more data: to reduce the size of possible statistical
fluctuations.
One might think that, once the analysis is
defined, it is just a matter of passing all the newly accumulated data
through those selection criteria in order to extract the type of events
we want to study. However, producing new results requires an incredible
number of checks and cross-checks.
The
analysis technique works as follows: a theoretical model is used to
predict what phenomena and particles might be seen, and experimental
physicists estimate what their detector response would be to such
events, using complex simulation methods. They do this first for all
known processes, so that they can predict the various expected types of
events that will come out of the LHC. These simulated events look just
like the events collected in the detectors, except they are generated
using all our knowledge of what can be produced when protons collide in
the LHC. Then the experimentalists determine a series of criteria for selecting new physics, partly defined using simulations. The selection criteria are designed for the sole purpose of spotting a needle in a field full of haystacks. For this, physicists study in detail the characteristics of possible interesting events (such as the Higgs boson), comparing these characteristics with those of known processes. At this stage, the name of the game is to isolate the signal from all other types of events, which physicists refer to as background. Most of the time, the background constitutes the bulk of all collected events. The final step is to compare the simulations of the known processes that survive the selection criteria to the collected data set. In some cases, comparison with simulations might not be necessary, and physicists may just need to subtract potential Higgs signals from the background directly inferred from the actual data. |
The more data are collected, the more precise these comparisons get,
making the result more significant. In the end, the goal is to produce
absolutely trustworthy results, excluding flaws, bugs and oversights.
- What are the next steps?
The data recorded so far in 2012 have not been completely analysed, and
the LHC is still taking data. Further analysis is needed and ongoing.
Despite the strong evidence for its existence, the properties of the
Higgs boson need to be explored and understood.
As the particle is identified and studied more completely, the physics models will have to be updated (also read Question 9).
In the meantime, the LHC will continue its scientific programme of
which the Higgs is only one item. By exploring the world of infinitely
small particles, physicists hope to provide answers to the origin and
fate of our universe. What happened just after the Big Bang? Why did
matter dominate over anti-matter when, in laboratory settings, they are
created in equal amounts? Finding out what dark matter is made of is
certainly high on the LHC agenda, even if popular models such as
supersymmetry have not manifested themselves yet, despite all our
attempts at unveiling them. What would you say if you found out we do
not live in a four-dimensional world (three dimensions of space and one
of time), but rather one containing extra hidden dimensions? There are
enough strange, puzzling questions and even stranger possible answers to
blow your mind!
In particle physics as in other research
fields, scientists will continue to study how the Universe works. With
the Higgs, the Universe has disclosed just one of its numerous
mysteries.
- What is the impact of such a Higgs boson on the current description we use for the Universe?
The Higgs boson will complete our description of the visible matter in
the Universe, and of the fundamental processes governing the Big Bang
since it was a trillionth of a second old. The Higgs boson may have
played a role in generating the matter in the Universe, and may be
linked to dark matter. It may even provide a clue how the Universe
inflated to its present size. On the other hand, the Higgs boson is a
very different particle from the others we know, and poses almost as
many questions as it answers. For example, what determines the mass of
the Higgs boson and the density of dark energy? According to
conventional ideas, both should be much larger than their observed
values. The quest continues.
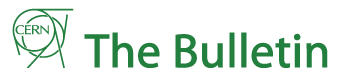
La imagen muestra las huellas de dos fotones de alta energía, en un
experimento del tipo utilizado para determinar la presencia de
partículas subatómicas.
Sigue el misterio sobre nueva partícula descubierta por el CERN
La institución europea reveló que encontró una partícula "consistente con el bosón de Higgs", aunque no pueden confirmar que se trate del buscado elemento, clave para entender la formación del universo.
GINEBRA.- El Centro Europeo de Física de Partículas (CERN)
anunció hoy el descubrimiento de una nueva partícula que podría ser el
buscado "bosón de Higgs", aunque todavía no puede confirmarlo con
certeza científica.
Miles de científicos del CERN y otros laboratorios del mundo trabajan desde hace décadas en la búsqueda de esa partícula, que es la clave para entender mejor la formación del universo.
Los primeros indicios del enigmático descubrimiento se conocieron el martes, cuando un video publicado por error en el sitio del CERN anunció la detección de la partícula, que tendría una masa cercana a la del bosón de Higgs.
"Hemos observado una nueva partícula y tenemos indicios muy fuertes de que hay algo", señaló en el video el físico Joe Incandela, de la Universidad de California y portavoz de uno de los dos equipos que buscan la partícula elemental en el Cern, lo que despertó alerta entre los científicos de todo el globo.
Esta mañana, el CERN anunció que encontró una nueva partícula, con certeza 4,9 sigma. Joe Incandela explicó que "sabemos que debe ser un bosón y que sería el más pesado encontrado hasta ahora. Las implicancias son muy significativas y es precisamente por esto que debemos ser extremadamente diligentes en todos nuestros estudios y confirmaciones".
Los datos publicados hoy fueron obtenidos en 2011 y 2012, aunque todavía hay resultados que no se han analizado. La información analizada coincide con el periodo más prolífico del Gran Acelerador de Hadrones (LHC).
Los próximos pasos, según publicó el CERN, es confirmar la naturaleza de la partícula encontrada; si es el bosón de Higgs o si es una partícula más exótica. Ambas alternativas son interesantes: una explicaría el origen de la materia del universo (que equivale al 4% de éste) y la otra podría dar luces sobre el 96% restante.
"Maldita" partícula
En el "modelo estándar" (teoría de la estructura fundamental de la materia elaborada en la década de 1960 para describir a todas las partículas y fuerzas del universo), el bosón de Higgs es considerado la partícula que brinda su masa a todas las demás.
Al intentar aislar los más pequeños componentes de la materia, los físicos descubrieron varias series de partículas elementales. Así, seis tipos de quarks (llamados "up" en inglés, lo cual significa "arriba", "down" o "abajo", "charm" o "encanto", "strange" o "extraño", "top" o "cima" y "bottom" o "fondo") forman parte de los componentes básicos o "ladrillos elementales" de la materia, al igual que el electrón y sus hermanos mayores, el muon y el tau, y tres tipos de neutrinos.
Estas 12 partículas interactúan entre ellas, por intermedio de mensajeros, llamados bosones. Uno de ellos es el fotón, que porta la radiación electromagnética, y otro el gluon, que brinda cohesión a los núcleos atómicos.
El fotón, que viaja a la velocidad de la luz, no tiene masa, característica que, según los científicos, no proviene de las partículas mismas.
En 1964, por deducción, el físico británico Peter Higgs postuló que existía el bosón que hoy lleva su nombre y que debía dar su masa a otras partículas.
"La idea es que hay partículas que chocan permanentemente con bosones de Higgs. Estos choques frenan su movimiento, que se vuelve más lento, y le dan la apariencia de una masa", explica el físico y filósofo Etienne Klein.
El especialista compara este fenómeno con un hombre que intenta pasar corriendo en medio de una multitud que "frena su carrera" y le hace aminorar su velocidad. También compara al campo de Higgs con una especie de pegamento en medio del cual se encontrarían relativamente adheridas las partículas, lo que se percibiría como masa.
Al bosón de Higgs se le llama "partícula de Dios", como consecuencia de un libro al que se le cambió el título. No obstante, el premio Nobel de Física Leon Lederman quería llamarlo "The Goddamn Particle" ("la partícula maldita"), por lo difícil que era encontrarla.
El editor sacó la terminación "damn" y lo llamó "The God Particle", ya que temía que la palabra "goddamn" fuera considerada insultante.
Miles de científicos del CERN y otros laboratorios del mundo trabajan desde hace décadas en la búsqueda de esa partícula, que es la clave para entender mejor la formación del universo.
Los primeros indicios del enigmático descubrimiento se conocieron el martes, cuando un video publicado por error en el sitio del CERN anunció la detección de la partícula, que tendría una masa cercana a la del bosón de Higgs.
"Hemos observado una nueva partícula y tenemos indicios muy fuertes de que hay algo", señaló en el video el físico Joe Incandela, de la Universidad de California y portavoz de uno de los dos equipos que buscan la partícula elemental en el Cern, lo que despertó alerta entre los científicos de todo el globo.
Esta mañana, el CERN anunció que encontró una nueva partícula, con certeza 4,9 sigma. Joe Incandela explicó que "sabemos que debe ser un bosón y que sería el más pesado encontrado hasta ahora. Las implicancias son muy significativas y es precisamente por esto que debemos ser extremadamente diligentes en todos nuestros estudios y confirmaciones".
Los datos publicados hoy fueron obtenidos en 2011 y 2012, aunque todavía hay resultados que no se han analizado. La información analizada coincide con el periodo más prolífico del Gran Acelerador de Hadrones (LHC).
Los próximos pasos, según publicó el CERN, es confirmar la naturaleza de la partícula encontrada; si es el bosón de Higgs o si es una partícula más exótica. Ambas alternativas son interesantes: una explicaría el origen de la materia del universo (que equivale al 4% de éste) y la otra podría dar luces sobre el 96% restante.
"Maldita" partícula
En el "modelo estándar" (teoría de la estructura fundamental de la materia elaborada en la década de 1960 para describir a todas las partículas y fuerzas del universo), el bosón de Higgs es considerado la partícula que brinda su masa a todas las demás.
Al intentar aislar los más pequeños componentes de la materia, los físicos descubrieron varias series de partículas elementales. Así, seis tipos de quarks (llamados "up" en inglés, lo cual significa "arriba", "down" o "abajo", "charm" o "encanto", "strange" o "extraño", "top" o "cima" y "bottom" o "fondo") forman parte de los componentes básicos o "ladrillos elementales" de la materia, al igual que el electrón y sus hermanos mayores, el muon y el tau, y tres tipos de neutrinos.
Estas 12 partículas interactúan entre ellas, por intermedio de mensajeros, llamados bosones. Uno de ellos es el fotón, que porta la radiación electromagnética, y otro el gluon, que brinda cohesión a los núcleos atómicos.
El fotón, que viaja a la velocidad de la luz, no tiene masa, característica que, según los científicos, no proviene de las partículas mismas.
En 1964, por deducción, el físico británico Peter Higgs postuló que existía el bosón que hoy lleva su nombre y que debía dar su masa a otras partículas.
"La idea es que hay partículas que chocan permanentemente con bosones de Higgs. Estos choques frenan su movimiento, que se vuelve más lento, y le dan la apariencia de una masa", explica el físico y filósofo Etienne Klein.
El especialista compara este fenómeno con un hombre que intenta pasar corriendo en medio de una multitud que "frena su carrera" y le hace aminorar su velocidad. También compara al campo de Higgs con una especie de pegamento en medio del cual se encontrarían relativamente adheridas las partículas, lo que se percibiría como masa.
Al bosón de Higgs se le llama "partícula de Dios", como consecuencia de un libro al que se le cambió el título. No obstante, el premio Nobel de Física Leon Lederman quería llamarlo "The Goddamn Particle" ("la partícula maldita"), por lo difícil que era encontrarla.
El editor sacó la terminación "damn" y lo llamó "The God Particle", ya que temía que la palabra "goddamn" fuera considerada insultante.
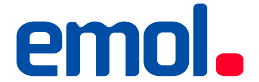
Qué es la Partícula de Biggs?
La Enciclopedia Wikipedia nos dice:
El bosón de Higgs es una hipotética partícula subatómica que constituye el cuanto del campo de Higgs. Tanto él como su campo asociado están relacionados con el origen de la masa de las partículas elementales. Al bosón de Higgs se le denomina habitualmente la partícula de Dios o la partícula divina, debido al libro La partícula divina: si el universo es la respuesta, ¿cuál es la pregunta? de Leon Lederman, ganador del premio Nobel de Física en 1988.
La existencia del bosón de Higgs fue predicha en 1964 como parte del mecanismo de Higgs, propuesto para explicar la masa de las partículas elementales del modelo estándar. En particular dicho mecanismo justifica la masa de los bosones vectoriales W y Z, que los diferencia de otros bosones que median interacciones fundamentales, como el fotón.
El bosón de Higgs recibe su nombre de Peter Higgs, que fue uno de los seis autores que en la década de 1960 desarrolló la idea del mecanismo ahora conocido por su nombre. Según el modelo estándar interacciona con todas las partículas con masa,2 no posee espín ni carga eléctrica o de color, y como su nombre indica es un bosón. Además es muy inestable y se desintegra rápidamente.
Debido a que su masa es presumiblemente muy grande, sólo puede ser detectado a altas energías en un acelerador de partículas. Este es uno de los objetivos principales del Gran Colisionador de Hadrones (LHC) del CERN, situado en Ginebra (Suiza), que comenzó sus experimentos en 2010. Anteriormente también se intentó en LEP (un acelerador previo del CERN) y en Tevatron (de Fermilab, situado cerca de Chicago en Estados Unidos).
El 4 de julio de 2012 se presentaron en el CERN los resultados preliminares de los análisis conjuntos de los datos tomados por el LHC en 2011 y 2012. Los dos principales experimentos del acelerador (ATLAS y CMS) anunciaron la observación de una nueva partícula «compatible con el bosón de Higgs», con una masa de unos 125 GeV/c2. El estudio de las propiedades de la nueva partícula, para confirmar si se trata efectivamente del bosón u otra posibilidad, necesita aún más tiempo y datos.1
Físico británico Higgs elogia hallazgo de bosón de Higgs
LONDRES, 4 jun (Xinhua) -- El físico británico Peter Higgs, quien
propuso la existencia del bosón de Higgs en 1964, elogió hoy los
hallazgos del Consejo Europeo para la Investigación Nuclear (CERN, por
sus siglas en francés) y los describió como una "ocasión para celebrar".
El CERN confirmó hoy con anterioridad que sus físicos observaron una nueva partícula consistente con el largamente buscado bosón de Higgs, una partícula subatómica teórica que se cree confiere la masa.
"Para mí es realmente increíble que esto haya ocurrido durante mi vida", dijo Higgs en una conferencia de prensa ofrecida en Ginebra.
Profesor emérito de la Universidad de Edimburgo, Higgs también señaló a través de la oficina de prensa de la universidad que los "científicos del CERN deben ser felicitados por los resultados de hoy, lo cuales son un gran logro para el Gran Colisionador de Hadrones y de otros experimentos que condujeron a esto".
"Estoy atónito por la increíble velocidad con la cual surgieron estos resultados. Son un testimonio del conocimiento de los investigadores y de las elaboradas tecnologías existentes", dijo el profesor en la declaración.
Por otra parte, el director de la Universidad de Edimburgo, el profesor Timothy O'Shea, dijo en una declaración que "estamos encantados con este significativo acontecimiento en la búsqueda del bosón de Higgs y felicitamos al profesor Peter Higgs".
O'shea elogió a Higgs, ahora de 83 años, porque "a lo largo de los años ha inspirado a muchos colegas y estudiantes, algunos de los cuales han participado en los experimentos del Gran Colisionador de Hadrones. Su legado seguirá inspirando a generaciones futuras de físicos en Edimburgo y más allá".
Los científicos han pasado décadas buscando el bosón de Higgs, frecuentemente llamado la "partícula de Dios", que se cree se desintegra casi de forma instantánea tras interactuar con otras partículas.
Guillermo Gonzalo Sánchez Achutegui
ayabaca@gmail.com ayabaca@hotmail.com
ayabaca@yahoo.com
Inscríbete en el Foro del blog y participa : A Vuelo De Un Quinde - El Foro!
4 comentarios:
El CERN califica de "histórico" el hallazgo de una nueva partícula
Esto supone un avance fenomenal "en nuestra comprensión de la naturaleza", declaró Heuer, quien calificó como "histórico" el hallazgo.
"El descubrimiento de una partícula consistente con el bosón de Higgs abre el camino para estudios más detallados, requiere mayores estadísticas, para escudriñar las propiedades de la nueva partícula. Además, es probable que haga la luz sobre otros misterios del Universo", comentó.
El CERN presentó hoy en una conferencia científica los resultados de los experimentos ATLAS y CMS, que buscan desde hace años de manera paralela, pero independiente, pruebas de la existencia de la partícula de Higgs, clave para entender la formación del Universo.
Con los resultados presentados hoy es prácticamente un hecho que la partícula anunciada corresponde a la descrita por Peter Higgs en los años sesenta, sobre la que reposa el Modelo Estándar de la Física de Partículas.
"Tenemos que sentirnos orgullosos y felices", agregó Heuer, para enseguida destacar que "esto es un inicio" y que "queda mucho trabajo" durante los próximos meses.
Los responsables del CERN decidieron ayer prolongar el funcionamiento del Gran Acelerador de Hadrones (LHC) durante tres meses más para recoger una mayor cantidad de datos y poder analizar las propiedades de la nueva partícula con más detalle y precisión.
El LHC debía apagarse en otoño, pero ahora seguirá al menos hasta finales de año para intentar confirmar con toda certeza que lo que se ha descubierto es el bosón de Higgs.
Esta partícula permite resolver diversas incertidumbres científicas sobre la formación del universo y aumenta la comprensión sobre la formación de la masa. EFE
Wilson
España colabora en hallazgo de la partícula que podría ser el bosón de Higgs........
Madrid, 4 jul (EFE).- Un equipo de investigadores del Consejo Superior de Investigaciones Científicas (CSIC) de España ha participado a través de los programas ATLAS y CMS en el hallazgo de una nueva partícula en el modelo estándar de física, que podría corresponder al bosón de Higgs, como se anunció hoy en Ginebra.
El Laboratorio Europeo de Física de Partículas (CERN) con sede en Ginebra anunció el descubrimiento de una nueva partícula, cuyos parámetros se podrían corresponder con el llamado bosón de Higgs, y España, uno de los principales contribuyentes a dicho laboratorio, participa con el 8,11% del total de las aportaciones para el ejercicio 2012, según el CSIC.
La participación de los grupos de investigación españoles en el acelerador de partículas LHC cuenta con el apoyo del Ministerio de Economía y Competitividad, a través del Programa Nacional de Física de Partículas y del Centro Nacional de Física de Partículas, Astropartículas y Nuclear (CPAN), proyecto Consolider-Ingenio 2010.
Los investigadores españoles, además de diseñar y construir varios subdetectores clave en la búsqueda de nuevas partículas en el acelerador LHC, participan de forma destacada en su operación y mantenimiento, así como en la recogida, procesado y análisis de las colisiones producidas en los experimentos.
En el proyecto de CMS, donde participan 3.275 científicos de 179 institutos en 41 países, están presentes los grupos experimentales del Centro de Investigaciones Energéticas, Medioambientales y Tecnológicas, Instituto de Física de Cantabria (centro mixto del CSIC y la Universidad de Cantabria), la Universidad de Oviedo y la Universidad Autónoma de Madrid.
Al igual que en el caso de ATLAS, la participación de los grupos españoles en actividades de análisis en CMS está muy diversificada. Todos los grupos participan activamente en la búsqueda del bosón de Higgs.
Con los resultados presentados hoy es prácticamente un hecho que la partícula anunciada corresponde a la descrita por Peter Higgs en los años sesenta, sobre la que reposa el Modelo Estándar de la Física de Partículas.
El director general del Centro Europeo de Física de Partículas (CERN), Rolf Heuer, confirmó el descubrimiento de esa nueva partícula, "consistente" con lo que se cree es el "bosón de Higgs".
Es un avance fenomenal "en nuestra comprensión de la naturaleza", declaró Heuer, quien calificó como "histórico" el hallazgo.
La confirmación de la detección del boson de Higgs permitiría resolver diversas incertidumbres científicas sobre la formación del universo y aumenta la comprensión sobre la formación de la masa.
En caso de que, tras realizar las pruebas estadísticas y de otro tipo aún necesarias, se tratara de otra partícula y no el bosón de Higgs, según indicaron los científicos del CERN, el hallazgo comunicado hoy sería igual de importante, pues abriría otras puertas a la física.
"El descubrimiento de una partícula consistente con el bosón de Higgs abre el camino para estudios más detallados, requiere mayores estadísticas, para escudriñar las propiedades de la nueva partícula. Además, es probable que haga la luz sobre otros misterios del Universo", comentó el científico.EFE
Leopoldo
El CERN anuncia el descubrimiento de nueva partícula, con gran probabilidad de ser el bosón de Higgs .....
Ginebra, 4 jul (EFEverde).- El Laboratorio Europeo de Física de Partículas (CERN) anunció hoy el descubrimiento de una nueva partícula, que podría ser el buscado "bosón de Higgs", aunque todavía no puede confirmarlo con certeza científica.
Miles de científicos del CERN y otros laboratorios del mundo trabajan desde hace décadas en la búsqueda de esa partícula, que es la clave para entender mejor la formación del universo. "Hemos observado un exceso de sucesos alrededor de una masa de unos 125
gigaelectronvoltios (GeV) con una significación estadística de 4,9 sigmas", dijo el físico Joe Incandela, portavoz del CMS, uno de los dos experimentos que buscan la partícula de Higgs.
Esto significa que la nueva partícula observada tiene las propiedades que se espera correspondan al "bosón de Higgs" y que esto ha podido observarse con una probabilidad de error equivalente a una en tres millones.
El CERN presentó en una conferencia científica en su sede principal los resultados obtenidos hasta el momento por el experimento CMS y ATLAS, en la víspera de una prestigiosa reunión de Física de Altas Energías en Australia. EFEverde
Juan
Conozca la importancia del descubrimiento de la ´Partícula de Dios´
Investigadores suizos han presentado uno de los descubrimientos más importantes de la historia, la partícula subatómica, conocida como la Partícula de Dios, que es pieza fundamental en la formación de la vida, luego del Big Bang de hace 13.700 millones de años.
Existe una teoría que explica la formación general del Universo denominada Modelo Estándar y que necesitaba de esta última partícula (denominado por Peter Higgs como bosón de Higgs), para ser del todo válida. Con el descubrimiento de esta partícula se ayuda, definitivamente, a comprender la organización del cosmos.
El Modelo Estándar es la mejor definición que ha encontrado la física sobre cómo se estructuran los elementos que forman el universo. Detalla la coexistencia de 12 partículas fundamentales, gobernadas por fuerzas básicas.
Los expertos creen que tras el Big Bang, el universo se convirtió en una gran sopa de partículas avanzando en distintas direcciones a la velocidad de la luz, sin ninguna masa. Cuando se dio la interacción con el campo de Higgs (campo de energía presente en todo el cosmos), ganaron masa y, con el tiempo, se formó el universo.
Corroborar el Modelo Estándar, o transformarlo, sería un paso hacia adelante de la física, que integre la materia oscura, la energía oscura y la fuerza de gravedad. Además, podría incrementar la posibilidad de la existencia de universos paralelos.
Investigador.......
Publicar un comentario